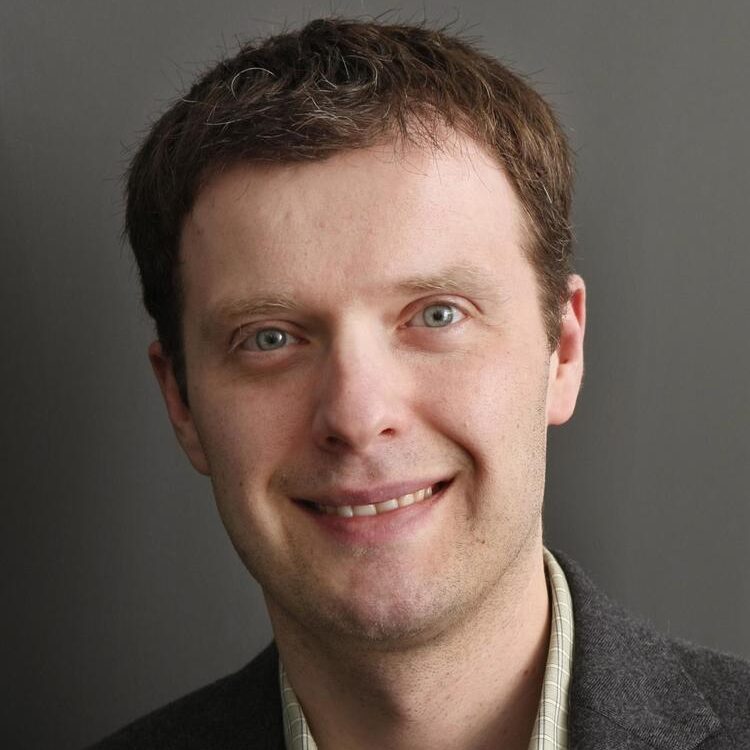
As a student in his ENE 431: Solar Energy Conversion course, I’ve had the privilege of learning from Professor Rand this semester. This course has challenged how I think about energy and prompted me to look more closely at the systems that power the world. What stood out to me wasn’t just the technology, but the sense of possibility it held. I found myself thinking more seriously about what the future of energy could look like, and who’s shaping it. That curiosity led me to interview Professor Rand for Now & Next, where we discuss his research in thin-film electronics and where he sees the field heading.
What’s Going On Now?
Shannon Yeow (SY): What excites you about working in thin-film electronics?
Barry Rand (BR): Thin-film electronics1 excite me because they allow us to create electronic systems that don’t directly compete with traditional silicon-based technologies. Instead, they access entirely different domains, enabling applications that silicon can’t easily reach, such as very large displays or next-generation solar technologies. While silicon currently dominates the solar industry, that may not always be the case. My research focuses on uncovering new properties of thin-film semiconductors and exploring how we can integrate them into real, commercially viable products.
SY: What are the most promising materials your lab is currently investigating, and how might they transform applications like solar energy?
BR: The first is the class of metal halide perovskites2, which we work on in my lab. These materials are especially relevant to the field of optoelectronics, in applications similar to LEDs or displays, but they are likely to be most impactful initially in solar photovoltaics3. In lab environments, the performance of perovskite solar cells has risen to rival that of traditional silicon solar cells. If progress continues in improving their stability and robustness, we may begin to see perovskites deployed at scale in photovoltaic systems. This would be a major step toward building a more sustainable energy ecosystem.
The second major class of materials is known as 2D materials: atomically thin, layered compounds that typically consist of just one to three layers of atoms. These materials are gaining importance as potential components of large-area electronic devices and next-generation electronics. What makes them especially promising is their ability to integrate relatively easily with conventional silicon-based systems, which could accelerate their adoption in the broader electronics industry.
SY: How do halide perovskites compare to current photovoltaic technologies, and what impact could they have on the future of solar energy?
BR: Most photovoltaic installations today are based on silicon solar cells, which are assembled into modules. Another class of photovoltaic technology is thin-film photovoltaics, where the dominant material is currently cadmium telluride (CdTe). Halide perovskites are a newer thin-film technology, like CdTe, but what makes them especially intriguing is that they don’t necessarily compete directly with silicon. CdTe and silicon are often seen as competitors in terms of which can deliver energy at the lowest cost, typically measured by the levelized cost of electricity (LCOE). This metric factors in both installation cost and efficiency over the device’s lifetime. Perovskites, however, offer a different opportunity: they can be integrated with silicon in a “tandem cell” configuration, where a perovskite layer is stacked on top of a silicon cell. This combination can achieve higher efficiency than either material alone. Historically, increased efficiency has been a key driver of adoption in solar technology. More efficient panels mean fewer are needed to produce the same amount of energy, reducing space and installation costs. So, it’s easy to imagine a future where a standard photovoltaic panel is composed of a silicon base with a perovskite layer on top, offering both high efficiency and cost competitiveness.
SY: Why is improving solar panel efficiency so important for the future of renewable energy infrastructure?
BR: I think that the idea of “making less do more” will always be a winning proposition, especially when it comes to solar energy. At the end of the day, solar installations take up space, and while the total solar resource across the surface of the Earth is enormous, the energy density at any given location is relatively low, especially when compared to fossil fuels. Looking ahead, the renewable energy grid4 of the future will need to be over-provisioned. That means building in excess capacity to account for periods of insufficient sunlight, wind, or other renewable sources. So there will inevitably be an overpopulation of panels or turbines to ensure energy reliability. This is where efficiency becomes critical. If we can generate more power with fewer or smaller devices, that reduces the land area needed for generation, which is valuable because we’ll also need space for energy storage systems, whether that’s hydropower, pumped storage, or electrochemical batteries. So, minimizing the footprint of solar infrastructure while maximizing output is not just desirable, it’s necessary for a sustainable and resilient energy grid.
What Comes Next?
SY: On a global scale, how do you see solar evolving to meet energy demands, especially in developing regions?
BR: One of the most promising aspects of the learning curve, or experience curve, in renewable energy is that as we deploy more systems, the cost of deployment consistently goes down. This means that more advanced economies, with higher GDPs, are effectively driving down the global cost of these technologies through scale and innovation. In doing so, they are indirectly enabling emerging economies to access and adopt renewable energy systems at lower costs, often cheaper than traditional fossil fuel options like coal or even nuclear power. This creates a powerful opportunity for leapfrogging. Just as many developing countries skipped over wired telephony and went straight to wireless communication, we could see a similar leap in energy technologies.
SY: What are the biggest engineering challenges that still stand in the way of widespread renewable energy adoption?
BR: I think what we really need is a better understanding of how to manage the intermittency of renewable energy resources. It’s not just about improving generation technologies, it’s about figuring out how to balance supply and demand more intelligently. That could mean deploying large-scale energy storage solutions, or developing smart demand response systems that can reduce or shift electricity consumption during times of low supply. In my view, many of the most impactful innovations will need to happen at the grid management level. Ultimately, land is limited, capital is limited, and the challenge lies in identifying the right combination of technologies for each location. There’s no one-size-fits-all solution.
SY: Is there a renewable energy solution you think is under-hyped or deserves more attention?
BR: I wouldn’t necessarily say it’s underhyped, but I do think there’s a crucial and enduring role for fuels in our future energy ecosystem. When combusted, fuels release a large amount of energy, and some sectors, like aviation, are especially dependent on that. If we want these systems to continue existing in a fossil-fuel-free world, we’ll need to develop carbon-neutral fuels that can still meet the energy demands of those applications. Of course, some sectors can be electrified. With better train infrastructure, short-haul air travel could even be rendered unnecessary in some regions. However, for long-haul aviation, carbon-free or carbon-neutral fuels may be essential. Identifying these hard-to-electrify sectors and developing viable fuel alternatives is still a challenge, and one that I believe requires more investment and research.
SY: What changes do you think we will see in energy and sustainability 10 years from now?
BR: I think we’re going to start seeing more real-world case studies that show what happens to grid reliability, grid composition, and the lives of local inhabitants when there’s a substantial deployment of intermittent renewables. These case studies will vary based on regional specifics, but some will highlight challenges or failures, which will be valuable learning opportunities. Right now, many systems rely on fossil fuel backups. Going forward, I think we’ll start to see more examples of how to effectively manage grids with a high composition of intermittent renewables, without needing to fall back on fossil fuels. Once we have enough of those examples, the challenge of global deployment will shift. It will become less about fundamental engineering and more about political will and economic feasibility. I think over the next two decades, we’ll see this transition play out in really interesting and varied ways around the world.
SY: What makes you hopeful about the future of engineering and climate innovation?
BR: One thing I’ve noticed this semester is that the class I’m teaching, ENE 431: Solar Energy Conversion, has the largest enrollment I’ve ever had, with 38 students. That gives me a lot of hope. It suggests there’s a growing interest in understanding solar energy and renewable technologies. If that kind of enthusiasm on this campus is at all representative of a broader trend—a sort of bellwether for what’s happening at other universities around the world—then I believe there’s real momentum building within society. With that momentum, I’m optimistic that we’ll see these types of technologies become central to how we source energy in the future.
Interview responses have been edited for clarity and length.
—
Speaking with Professor Rand reminded me how engineering innovation is never about one big solution, but about many small, smart, and intentional steps. From organic semiconductors to tandem solar cells and grid management strategies, his research points toward a more efficient and sustainable future. As someone still exploring where I fit into this space, our conversation deepened my appreciation for the people driving change behind the scenes. I hope this interview sparked new insights and inspiration. I can’t wait to share more current events, breakthroughs, and trends in the next edition of Now & Next.
— Shannon Yeow, Engineering Correspondent
Glossary
- Electronic devices that use thin layers of materials deposited onto a substrate, enabling flexible, lightweight, and cost-effective electronics. ↩︎
- A yellow, brown, or black mineral consisting largely of calcium titanate. ↩︎
- Relating to the production of electric current at the junction of two substances exposed to light. ↩︎
- An interconnected network that delivers electricity from power plants to consumers. ↩︎